In Summary
- A high-risk area (HRA) is an area designed to high standards of hygiene where practices relating to personnel, ingredients, equipment and environment are managed to minimise microbiological contamination of a ready-to-eat product comprising of cooked (or finished) ingredients.
- Generally, implementing controls between the HRA and other parts of the plant (such as areas with raw product) is considered good practice.
- A table is provided below identifying good practices for implementing HRA controls, with the reasons provided.
- Notable controls include; staff movement restrictions, restricting water use and cleaning and sanitising equipment.
Definition of a high-risk area (HRA)
A HRA is defined as an area designed to high standards of hygiene where practices relating to personnel, ingredients, equipment and environment are managed to minimise microbiological contamination of a ready-to-eat (or ready-to-reheat) product comprising of cooked ingredients (e. g. hot smoked fish). Good manufacturing practice (GMP) dictates that hot smoked fish should be manufactured in a HRA, physically separated from uncooked and otherwise raw materials and also the final packaged product. Table 1 presents a list of observed good practices relating to a HRA which were observed by Rotariu et al. (2014) and Thomas et al (2014), predominantly in larger processing plants.
Table 1: Good practices observed for plant segregation
Identified good practice | Reason for practice |
---|---|
Existence of physical separation between:
|
Avoidance of cross contamination of the final product from potential Listeria sources (raw product, personnel, environment, packing material) |
Existence of a formally defined high risk area for cooked product | |
Existence of staff movement restrictions between the “clean” and the “dirty end” of the process (e. g. where there are raw, uncooked materials). Change of overalls, PPE, and handwashing before moving between different areas of the plant. | Avoidance of cross contamination to the final product from potential Listeria sources coming from the raw product side of production and transferred via staff. |
PPE of different colours for different processing areas. | Make inappropriate movements visibly obvious. |
PPE of different colours for different workers. | Make staff working inappropriately visibly obvious (e. g. cleaning staff operating processing equipment and thereby contaminating control panels). |
An elevated floor height of around 1.5 metres in HRA compared with the rest of the plant. | The floor helped enforce controlled movements of staff, products and equipment. For example, finished, packed product was dropped from conveyors into Dolavs (large plastic crates) and could not inadvertently return to the high-risk area. |
Automatic disinfectant boot scrubbers at the start of a long (~25m) corridor that was physically separated but exited into high risk so that boots had dried (i. e. contained little or no water) before they reached the HRA. | Little or no water in high risk helps prevent the establishment of L. monocytogenes. |
Use of visible or physical barriers. | A number of FBOs felt a physical barrier such as a bench where employees left their shoes on one side and put work boots on, on the other side of the barrier was helpful in reminding employees they had entered a controlled area. One manufacturer had installed an array of bright bluish fluorescent tubes (non-UV) at the entrance to the HRA as a visible reminder of the area’s status. |
Staff movement restrictions
In addition to the observations of Hutchison et al. (2014), there are multiple reports that L. monocytogenes can be isolated from PPE such as the gloves and aprons of workers involved in the processing of fish and seafood (Rørvik et al. 1997; Autio et al. 1999; Tompkin et al. 1999; Thimothe et al. 2002; Gudbjörnsdóttir et al. 2004; Hansen et al. 2006; Pagadala et al. 2012; Jami et al. 2014). Positive isolations have been reported to range from 5% to 100% of the samples collected. In addition, Rørvik et al. (1997) report that job rotation, where workers undertake different tasks in different areas of a processing plant, is a risk factor for L. monocytogenes isolation from final product fish. Thus, it is well established that process workers can be a source of L. monocytogenes contamination.
Although well established (Aalto-Araneda et al. 2019), the importance of workers as fomites [glossary entry] is underscored by papers that have undertaken mathematical modelling of cross contamination from workers within fish processing (Ivanek et al. 2004). A model was developed, and its predictions validated using real world observations. The model and corresponding real-world observations showed that a simple intervention such as changing gloves with greater frequency could significantly reduce the within lot prevalence of L. monocytogenes-contaminated final product fish (Ivanek et al. 2004). Furthermore, the decontamination, rather than replacement, of gloves has also been shown to be beneficial (Liu and Su 2006). Acidic EOW (electrolysed oxidising water) reduced and could eliminate L. monocytogenes contamination on a variety of latex and nitrile disposable and reusable gloves used by seafood processing workers (Liu and Su 2006).
Restricting the use of water
It is also well established that L. monocytogenes is commonly isolated from processing areas that are frequently wet (Tompkin et al. 1999; Tompkin 2002; Carpentier and Cerf 2011; Jami et al. 2014). These include chillers and air-conditioned sections of the factory if there is mixing between chilled and warm, moist air. The air released from an oven into a chilled HRA (high risk area) can be particularly problematic. Carpentier and Cerf (2011) summarise the issues with water as “Minimal use of water, and no water use in areas where the product is exposed, should be the rules. In these areas, water should only be applied during cleaning and disinfection and drying should take place before the next work shift. Such rules have been successfully applied in plants to prevent product contamination with L. monocytogenes”. Floors and drains, which are frequently wet, are also a well acknowledged reservoir for L. monocytogenes in all food processing plants (Berrang et al. 2010; Fox et al. 2014; Dzieciol et al. 2016). The issue is a concern not only for fish (Tompkin et al. 1999). There are a number of mechanisms that can spread contamination from drains. In separate, but closely-related studies, (Berrang and Frank 2012) and (Saini 2012) inoculated Listeria into floor drains to final concentrations of 104 cfu/cm2 bound to the wall of the drain and 108 cfu/ml of liquid in the drain. The drains were then hosed with a burst of medium pressure (~70kPa) tap water for two seconds and any airborne Listeria generated by the water impact were captured using impaction samplers (Berrang and Frank 2012) or stainless steel coupons (Saini 2012) and also settle plates placed in the areas around the drains. Both studies had robust replication and the both sets of authors observed that Listeria spp. was recovered at distances of up to 4 m from the sprayed drain and from walls at heights as high as 2.4 m above the floor. Hosing the drains with a medium-pressure hose created aerosols of Listeria. Food that was present during the hosing, and food that was brought in 10 minutes afterwards (Berrang and Frank 2012) were both contaminated with Listeria as the aerosolised bacteria settled back out of the air onto horizontal surfaces (Saini 2012; Berrang et al. 2013; Dzieciol et al. 2016). Saini (2012) also observed transfer from drains during simulated cleaning and disinfection. When water is used for cleaning and disinfection of a HRA (high risk area) [Hyperlink to glossary entry], there is benefit in drying the residual moisture before the commencement of processing (Tompkin et al. 1999; Tompkin 2002; Carpentier and Cerf 2011). Tompkin (2002) reports that an effective strategy to contain an accidental spillage of water in an HRA is to apply citric acid crystals to the affected area. Some stress-hardened L. monocytogenes strains can become acid tolerant, but most L. monocytogenes are unable to multiply at pH 4.5 or below (Koutsoumanis et al. 2003).
Equipment cleaning and sanitation
There are many publications that have demonstrated persistent strains of L. monocytogenes can become established in a fish-processing plant and repeatedly isolated from the plant environment and manufactured products for months and years (Autio et al. 1999; Mędrala et al. 2003; Ferreira et al. 2014; Harrand et al. 2020). It is a key strategy for the control of L. monocytogenes that any equipment that is present in a HRA is effectively cleaned and sanitised. Given the previous comments relating to the minimal use of water in a HRA, many larger processors remove equipment into adjacent anterooms for cleaning, sanitation and drying before returning the disinfected equipment to the HRA.
There is debate as to whether L. monocytogenes persistence is linked with an ability to form a biofilm, [glossary entry] if it is a fortuitous chance or if both mechanisms can result in long-term L. monocytogenes establishment. In general, L. monocytogenes is exposed to a range of sanitising chemicals in food processing areas, sometimes at concentrations below those recommended by manufacturers (Allen et al. 2016). Allen et al. (2016) also considered that sublethal exposures may last for long periods, are frequently repeated, and potentially a route that may promote increased resistance over time to quaternary ammonium and phenolic based sanitizers along with the promotion of plant residency and persistence. However, the issue is not clear cut. In general, sanitiser resistance does not correlate with the ability of L. monocytogenes strains to persist in a processing plant (Ferreira et al. 2011). Carpentier and Cerf (2011) asserted that L. monocytogenes persistence is primarily a random accident because there have been no genes identified within L. monocytogenes that confer persistence, but niches exist in food industry premises and equipment where L. monocytogenes persist (Wang et al. 2015). Having comprehensively reviewed a large body of pertinent literature, Martínez-Suárez et al. (2016) concluded that local environmental conditions in plants create persistent niches [glossary entry] fortuitously by blocking full exposure to disinfectants.
Effective cleaning and sanitation are key to preventing persistent L. monocytogenes. The first record of L. monocytogenes as a food pathogen was reported in the 1980s. There are still processing equipment in operation that predate L. monocytogenes as a foodborne pathogen. Difficult or impossible to access areas can exist on such equipment that was not hygienically designed (Carpentier and Cerf 2011). The classic L. monocytogenes harbourage locations are hollow parts such as conveyor rollers, duct support rods and unpolished welds on processing equipment that contain holes (Tompkin et al. 1999). Carpentier and Cerf (2011) provide visual evidence that the natural degassing of freshly deposited plastics of the type used to form conveyor belts can leave bubble holes that can be colonised by L. monocytogenes. Other potential persistent locations of note include crevices and cracks or otherwise damaged flooring and drains (Tompkin 2002).
In general, effective cleaning and sanitation can be achieved from suspected contaminated (or positive tested) equipment by dismantling the machinery and cleaning and sanitising the components individually. The components can be sampled to confirm they are free from L. monocytogenes. Although such an approach is normally adequate, occasionally there may be components with design deficiencies that are impossible to sanitise using chemicals. For small pieces of equipment, an application of heat using a hot water (80oC) bath with detergent and high frequency sound (e.g. sonication) may be effective (Tompkin 2002). For larger equipment that can be moved, sensitive components such as electronics and greased cogwheels can be disassembled and removed and heat applied to the equipment in an industrial oven. Moist heat is more effective than dry heat. Tompkin (2002) advises that if equipment cannot be moved into an oven, it can be covered in a heat resistant tarpaulin and steam (e. g. from a wallpaper stripper specifically bought for the purpose) can be introduced from the bottom of the equipment. A target of at least 80oC should be attempted, measured by thermocouples and the temperature should be held for at least 20 min. Prior to reassembly, lubrication of sanitised components can be applied as grease containing anti-listerial sodium benzoate (El-Shenawy and Marth 1988).
Unless special measures are used, HCA (High care areas) and HRA (high risk areas) [Hyperlink to glossary entries] are subject to a near constant bombardment of fomites that are potentially contaminated with L. monocytogenes (Tompkin 2002; Carpentier and Cerf 2011). How any potentially contaminated objects entering HCA/HRAs are controlled is a matter for a FBO to decide. The following strategies and interventions are not taken from peer reviewed scientific literature, but were considered by plant technical managers to be effective in reducing L. monocytogenes detections in smoking plants in the UK.
- Alarms were fitted to fire doors in HCA/HRA to stop workers going outside to smoke in PPE and footwear and returning to the HCA/HRA without re-dipping their boots.
- All packaging was slid into the HCA/HRA down a ramp. The packaging was ordered from the manufacturer as double bagged and the outer bag was removed immediately before being slid into the HCA/HRA.
- L. monocytogenes is not significantly transferred by airborne means (aside from aerosol splash). However, one plant found benefit in blowing filtered air at ambient temperature into ovens after baking. The practice removed warm, moist air from the oven and helped prevent condensation when the air in the oven was mixed with chilled air in the HCA/HRA.
- Copper drain covers reduced the number of detections of L. monocytogenes from drains compared to the same drains when covered with steel. Copper has widely acknowledged antimicrobial properties (Vincent et al. 2016).
- Copper wire was stapled to the inside of chiller door seals to help prevent L. monocytogenes growth.
- Paper towel, liquid soap, hand gel and gloves dispensers were emptied prior to wet cleaning of the plant environment and restocked afterwards.
- The installation of extra alcohol gel dispensers around the processing area including door handles that dispensed alcohol gel when used (below) reduced L. monocytogenes detections from the plant environment. Lappi et al. (2004) report L. monocytogenes isolations from door handles in fish smoking plants.
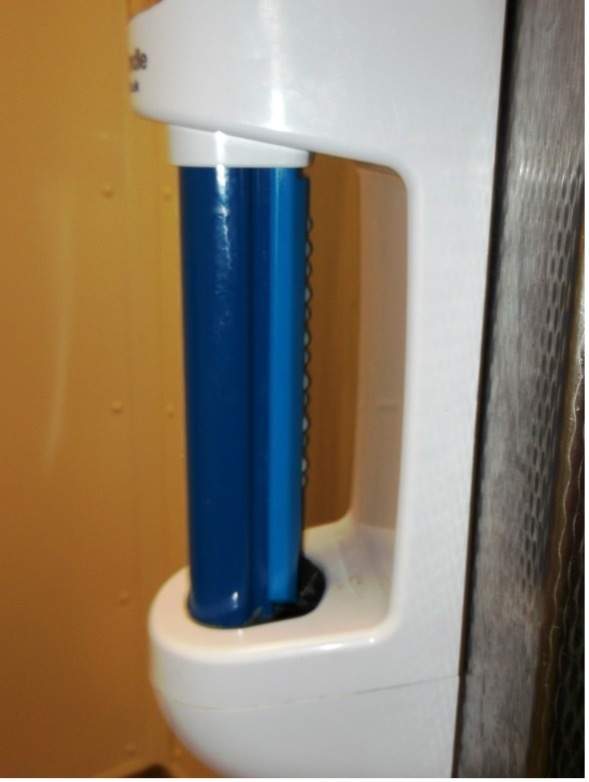